Pulmonary Contusion and Flail Chest, Management of
Published 2012
Citation: J Trauma. 73(5):S351-S361, November 2012
Authors
Simon, Bruce MD; Ebert, James MD; Bokhari, Faran MD; Capella, Jeannette MD; Emhoff, Timothy MD; Hayward, Thomas III MD; Rodriguez, Aurelio MD; Smith, Lou MD
Author Information
From the Division of Trauma and Critical Care (B.S., T.E.), University of Massachusetts Medical Center, Worcester, Massachusetts; Department of Emergency Medicine (J.E.), Dartmouth Hitchcock Medical Center; Department of Trauma, Burns, and Critical Care (F.B.), John H. Strogen Jr. Hospital of Cook County, Chicago, Illinois; Altoona Regional Medical Center (J.C.), Altoona, Pennsylvania; Indiana University/Wishard Memorial Hospital (TH), Indianapolis, Indiana; Sinai Hospital of Baltimore (A.R.), Baltimore, Maryland; Department of Surgery (L.S.), University of Texas.
Address for reprints: Bruce J Simon, MD, UMass Memorial Medical Center, Worcester, MA.
Statement of Problem
Thoracic injury and the ensuing complications are responsible for as much as 25% of blunt trauma mortality.[1] Pulmonary contusion (PC) in turn is the most common injury identified in the setting of blunt thoracic trauma, occurring in 30% to 75% of all cases.[1] Isolated PC may occur consequent to explosion injury, but most patients with multiple injuries have concurrent injury to the chest wall.[2] Conversely, flail chest (FC), the most severe form of blunt chest wall injury with mortality rates of 10% to 20%, is typically accompanied by significant PC.[3–7] While injuries to the chest wall itself may rarely be the primary cause of death in patients with multiple injuries, they greatly impact management and the eventual survival of these individuals.[8] In some series, most of the severe lung contusions that require ventilatory support (85%) are associated with severe bony chest wall injury.[9]
Despite the prevalence and recognized association of PC and flail chest (PC-FC) as a combined, complex injury pattern with interrelated pathophysiology, the mortality and short-term morbidity of this entity have not improved during the last three decades.[1] Advances in diagnostic imaging and critical care have also failed to impact on outcome.[1] In addition, there may be significant long-term morbidity associated with both PC and FC, the true extent of which remains unclear.[10][11] This injury constellation particularly affects the elderly who constitute approximately 10% of the cases but consume 30% of clinical resources.[9]
The unchanging mortality and morbidity of PC-FC have been attributed to a misunderstanding of the associated pathophysiology and a lack of scientifically proven successful management guidelines.[1] Consequently, significant controversy and a wide range of management philosophies exist, particularly as related to fluid management and ventilatory support.[5][7][12–29]
Questions to be Addressed
This evidence-based review will identify the extent and quality of scientific support for the management decisions in regard to the following questions:
- What are the appropriate principles of fluid management for patients with PCs?
- On ventilatory support:
- When is mechanical ventilation indicated for PC-FC?
- Is there a role for noninvasive ventilation?
- What is the optimal mode of ventilation for severe PC and/or FC?
- Is there a role for surgical fixation of FC injuries?
Process
A computerized search was conducted of the MEDLINE, Embase, and Cochrane databases for North American and European English-language literature for the period from 1966 through June 30, 2005. The initial search terms were pulmonary contusion, flail chest, rib fractures, chest injuries, and thoracic injuries. This search initially yielded 91 articles. An additional 39 works were obtained from the references of these studies yielding a total of 130 articles. Thirty-eight of these articles were excluded as being case studies, reviews, letters, or otherwise irrelevant to the questions being asked. The remaining 92 studies were reviewed, graded, and listed in the evidentiary table.
For the 2011 update of the guideline, a similar search was performed from July 1, 2005 through June 30, 2011. In this case, 85 articles were initially identified yielding 37 studies appropriate for grading by the same criteria.
The practice parameter workgroup for PC-FC consisted of eight trauma surgeons, three of whom were also trained and certified as thoracic surgeons. All studies were reviewed by two committee members and graded according to the standards recommended by the EAST Ad Hoc Committee for Guideline Development. Grade I evidence was also subgraded for quality of design using the Jadad Validity Scale published in Controlled Clinical Trials in 1996.[30] Any studies with conflicting grading were reviewed by the committee chairperson, as were all Grade I studies. Recommendations were formulated based on a committee consensus regarding the preponderance and quality of evidence.
Recommendations
Level 1
There is no support for Level 1 recommendations regarding PC-FC.
Level 2
Trauma patients with PC-FC should not be excessively fluid restricted but rather should be resuscitated as necessary with isotonic crystalloid or colloid solution to maintain signs of adequate tissue perfusion. Once adequately resuscitated, unnecessary fluid administration should be meticulously avoided.
- A pulmonary artery catheter may be useful to avoid fluid overload during resuscitation.
- Obligatory mechanical ventilation in the absence of respiratory failure solely for the purpose of overcoming chest wall instability should be avoided.
- Patients with PC-FC requiring mechanical ventilation should be supported in a manner based on institutional and physician preference and separated from the ventilator at the earliest possible time. Positive end-expiratory pressure (PEEP)/continuous positive airway pressure (CPAP) should be included in the ventilatory regimen.
- The use of optimal analgesia and aggressive chest physiotherapy should be applied to minimize the likelihood of respiratory failure and ensuing ventilatory support. Epidural catheter is the preferred mode of analgesia delivery in severe FC injury (see EAST PMG Analgesia in Blunt Thoracic Trauma).
- Steroids should not be used in the therapy of PC.
Level 3
- A trial of mask CPAP should be considered in alert, compliant patients with marginal respiratory status in combination with optimal regional anesthesia.
- There is insufficient evidence to prove the effectiveness of paravertebral analgesia in the trauma population. However, this modality may be equivalent to epidural analgesia and may be considered in certain situations when epidural is contraindicated.
- Independent lung ventilation may be considered in severe unilateral PC when shunt cannot be otherwise corrected owing to maldistribution of ventilation or when crossover bleeding is problematic.
- High-frequency oscillatory ventilation (HFOV) has not been shown to improve survival in blunt chest trauma patients with PC but has been shown to improve oxygenation in certain cases when other modalities have failed. HFOV should be considered for patients failing conventional ventilatory modes. The appropriate triggers for institution of HFOV have not been defined.
- Diuretics may be used in the setting of hydrostatic fluid overload as evidenced by elevated pulmonary capillary wedge pressures in hemodynamically stable patients or in the setting of known concurrent congestive heart failure.
- Although improvement has not been definitively shown in any outcome parameter after surgical fixation of FC, this modality may be considered in cases of severe FC failing to wean from the ventilator or when thoracotomy is required for other reasons. The patient subgroup that would benefit from early “prophylactic” fracture fixation has not been identified.
- There is insufficient clinical evidence to recommend any type of proprietary implant for surgical fixation of rib fractures. However, in vitro studies indicate that rib plating or wrapping devices are likely superior to intramedullary wires and these should be used as the preferred fixation device.
- Self-activating multidisciplinary protocols for the treatment of chest wall injuries may improve outcome and should be considered where feasible.
Scientific Foundation
Historical Background
Before the 20th century, the entity of PC had rarely been described, and its clinical significance was not recognized. During World War I, significant numbers of battlefield dead were noted to be without external signs of trauma and postmortem studies revealed lung hemorrhage.[31–33] Subsequently, the critical study during this conflict identified PC as the major clinically significant effect of concussive force.[34] This concept was confirmed during World War II by studies of animals placed at varying distances from explosive charges.[35–38] It was also first noted in military studies at that time that the contused lung produces more than its normal amount of interstitial and intra-alveolar fluid.[39] Aggressive fluid resuscitation was cited as a key factor in precipitating respiratory failure after blunt thoracic trauma.[40] Further studies during the Vietnam War laid the basis for the current philosophies in treatment of PC.[41–43] In a study of combined pulmonary and chest wall injury Reid and Baird[44] were the first to propose that parenchymal contusion rather than bony thoracic injury was the main factor in respiratory compromise.
Similarly, until the 1960s, the paradoxical movement of the FC component was believed to be the cause of respiratory compromise in blunt chest wall trauma.[39][45] It was presumed that this “Pendelluft” caused deoxygenated air to shunt back and forth to the healthy lung, rather than being exhaled, resulting in hypoxia. Consequently, treatment was aimed at correcting the paradoxical movement through a variety of methods including external fixation[46] and internal fixation by either surgical repair[47] or positive-pressure ventilation.[12][48] It was not uncommon to electively maintain patients on ventilatory support until bony union had occurred.[48] It is currently believed that the underlying lung contusion is a major cause of respiratory compromise with the bony chest wall injury creating the secondary problems of pain and splinting.[44] Contemporary practice has therefore been directed at addressing these issues[6][13][49]
Pathophysiology
The local pathophysiology of injured lung was first delineated by animal studies in the 1970s. Oppenheimer et al.[50] studied clinical behavior and pathologic findings in Class I study of contused dog lung. He identified contusions as lacerations to lung tissue, which leaked blood and plasma into the alveoli. He noted reduced compliance resulting in reduced ventilation per unit volume and increased shunt fraction. Other studies identified thickened alveolar septa in contused lung with consequent impaired diffusion.[51][52] Fulton et al.[14] defined the significant and progressive decrease in PO2 values in contused dog lung during a 24-hour period. An increase in pulmonary vascular resistance and consequent decrease in blood flow was noted in the contused lung. In other studies, these changes were not altered by the concurrence of FC injury.[53] In a small observational study of blunt trauma patients, Wagner et al.[54] also noted increases in pulmonary vascular resistance in proportion to contused volume and thought that this acted as a compensatory mechanism to minimize shunt fraction.
The effects of contusion on uninjured lung have also been recently elucidated through animal studies. Davis et al.[55] performed an elegant Class 1 study of a porcine model of blunt chest trauma. Unilateral chest trauma produced an early rise in bronchoalveolar lavage (BAL) protein on the injured side as well as a delayed capillary leak in the contralateral lung. Similarly, Hellinger et al.[51] showed that uninjured lung, both ipsilateral and contralateral, developed thickened septa, increased vacuolation and edema during an 8-hour period after injury. Although this occurred to an extent lesser than in injured lung, the findings were statistically significant compared with controls (p < 0.01) Moreover, in this study, BAL showed an increase in neutrophils (PMNs) in contused lung and ipsilateral and contralateral uninjured lung compared with controls. Local and systemic complement levels (terminal complement complex) increased and C3 complement decreased to a statistically significant level. More recently Perl et al.[56] showed that in the presence of experimental PC in mice, not only systemic inflammatory cytokines were increased, but also systemic cellular immunity was suppressed, and the survival of a distant septic challenge was significantly impaired compared with sham controls.
Consequently, high-grade evidence from animal studies indicated that PC is not merely a localized process but probably has global pulmonary and systemic effects relating to cytokine expression and immunosuppression when occurring in a sufficient portion of the lung.[56–59] Table 1 summarizes the reported physiologic effects of lung contusion. Reviewed literature is graded and summarized in the evidentiary tables.
Outcome
Numerous studies have addressed the outcome of PC-FC but have had difficulty in separating the effects of the chest wall and parenchymal components.[60–63] In terms of mortality, it remains controversial whether this constellation of thoracic injury is a direct cause of death[64] or merely a contributor in the setting of multiple injuries[3][65][66] In separate reviews, Clark and Stellin[65] both noted that central nervous system trauma was the most common associated injury. Few deaths in these retrospective studies (n = 144; n = 203) were caused by pulmonary failure per se but rather to brain injury and shock. While Relihan and Litwin[66] agreed that associated brain injury was the most common cause of death in patients with FC, his review (Class III, n = 85) indicated that complications of the pulmonary injury were contributory at least half the time. Flagel et al.[67] has similar findings in a large retrospective review, which showed that while length of stay (LOS) and mortality correlated with number of rib fractures, death seemed to be caused by other injuries or their complications. Conversely, Kollmorgen et al.,[64] in a retrospective review of 100 trauma deaths among patients with PC, thought that 70% of the deaths were caused by the lung injury or pulmonary failure primarily.
As far as morbidity is concerned, the need for ventilator dependence and the length of this dependence has been shown to correlate with the total contusion burden in terms of the percentage of lung volume, the number of rib fractures, overall injury severity score, age, and A-a gradient[60][62][68][69]
The long-term outcome of FC injury was first addressed in the 1980s by several workers.[11][70] In a retrospective review, Landercasper et al.[70] noted that 46% (n = 32) of patients with FC did not have normal chest wall expansion, 24% had obstructive changes on spirometry, and 20% had restrictive changes. Vital capacity had normalized in only 57%; 70% had long-term dyspnea, and 49% had persistent chest wall pain. The possible contribution of PC was not addressed, and computed tomographic (CT) scanning was not performed at this time. Similarly, Beal and Oreskovich[11] reviewed 20 patients with FC and a variety of associated thoracic injuries from 50 days to 730 days and also noted that the most common long-term problems were persistent chest wall pain, chest wall deformity, and exertional dyspnea. The etiology of the respiratory symptoms was not identified.
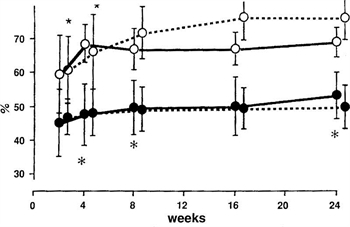
Figure 1.
In the 1990s, attempts were made to determine whether the FC, PC, or both components were responsible for the long-term disability, which is seen with the more severe injuries.[10][11][70][71] In a small (n = 18), but well-designed, blinded Class I study, Kishikawa et al.[10] followed up the pulmonary functions and radiographic findings of patients with PC-FC for 6 months. His group was trying to explain the persistent dyspnea often seen after blunt chest trauma. They noted that pulmonary function recovered within 6 months for patients without PC, even in the presence of severe residual chest wall deformity. However patients with PC had decreased functional residual capacity (FRC) and decreased supine PaO2 for years afterward. Figure 1, from the work of Kishikawa et al., shows the course of FRC for patients with PC with or without FC and with FC alone.
In an additional work by Kishikawa et al.,[71] 58% (n = 14 of 24) of contused lungs showed fibrosis on CT scan 1 year to 6 years after injury. The average spirometry for patients with contused lungs was 76% of normal versus 98% of normal for controls. Air volume measured by CT scan supported these findings. From these studies, it was concluded that the FC component causes short-term respiratory dysfunction, while the PCs are responsible for the long-term dyspnea, low FRC, and low PO2. The main cause of the persistent decreased air volume was thought not to be the residual thoracic deformity but rather the loss of pulmonary parenchyma by fibrosis of the contused lung. More recently, significant ongoing improvement in lung function after PC has been noted for up to 4 years by measurements of FeV1, FVC, TLC, CO2 diffusing capacity and p-exercise oxygen saturation.[61] Studies addressing the outcome of PC-FC are tabulated in the evidentiary tables.
Fluid Management
Present practice regarding type of quantity of fluid resuscitation for patients with multiple injuries and concurrent PC has been largely extrapolated from animal research or retrospective studies.[14–18][72] As early as 1973, Trinkle et al.[15] studied experimental right lower lobe PCs and noted that crystalloid resuscitation caused the lesions to be larger than did colloid use. Concurrent diuresis caused all lesions to decrease in size. However, when lesion size was corrected for lobe weight to body weight index, these results were not statistically significant. Moreover, in the 1970s, Fulton studied a dog model of PC and noted that fluid resuscitation increased the percentage of water in the contused lung over control groups resulting in “congestive atelectasis.” This effect was unchanged whether the animals were allowed to hemorrhage to shock before volume replacement or gradually resuscitated. Similarly, Richardson et al.[72] performed a well-designed randomized blinded (Class I) study of canine PC (n = 34). He noted that animals receiving lactated Ringer’s solution at various doses had declining oxygenation levels (PO2) and increased lung water when compared with those receiving plasma (p < 0.05). The authors concluded that colloid was superior to crystalloid for resuscitation in the setting of PC. However, in a single limb study of 109 human patients with PC, Bongard and Lewis[16] could not find a correlation between plasma oncotic pressure and oxygenation as determined by the PaO2/FIO2 ratio. He concluded that pulmonary dysfunction after contusion is unrelated to hemodilution by crystalloid. Finally, Richardson et al. retrospectively reviewed 86 patients with PC and found that mortality correlated with admission pulmonary function (PaO2/FIO2 < 300; p < 0.05) but not with the amount of fluid intravenously administered.
Decision for Ventilatory Support
As early as 1973, Trinkle et al.[15] showed that early intubation and application of PEEP decreased the size of experimental PC versus controls. Similarly, workers such as Shin provided some Class III evidence that progressive pulmonary deterioration in humans was lessened by immediate intubation and ventilation for every lung contusion.[12] Consequently, the de facto standard at that time for treatment of PC was obligatory mechanical ventilation. Yet, there were no credible data showing improved survival with this approach.[7]
Similarly, in the 1970s, it was thought that some form of stabilization of the mobile chest wall was the critical treatment of the FC component and that mechanical ventilation for “internal pneumatic stabilization” was the optimal way to achieve this regardless of the patient’s pulmonary function.[12][48] Evidence supporting this was mostly observational (Class II).[12][48] Workers such as Christensson et al.[12] thought that mandatory tracheostomy and 2 weeks to 3 weeks of positive-pressure ventilation would allow the chest wall to stabilize in a “favorable position.” Follow-up studies showed return of normal mechanics but nonventilated control groups were not used.
Trinkle et al.[7] was the first to raise the possibility that obligatory mechanical ventilation for FC was not necessary. In a small (n = 30) retrospective review with well-matched cohorts, the obligatory ventilation group had a longer hospital stay (22.6 days vs. 9.3 days, p < 0.005), a higher mortality (21% vs. 0%, p < 0.01), and a higher complication rate (23% vs. 2% p < 0.01) compared with the “selective group.” The “selective” group averaged only 0.6 ventilator days, indicating that the conservative management was often successful. Similarly, Richardson et al. studied 135 patients with isolated PC and 292 patients with PC-FC. Intubation was successfully avoided in 80% of patients with PC and 50% of patients with PC-FC. This study did not use matched cohorts, and the intubated patients were selected by failure of selective management. However, the study did demonstrate that most of the patients could be successfully managed without ventilatory support.
In a landmark work, Shackford et al.[13] performed a well-constructed case-control study (Class II) of selective ventilatory support with the end points of treatment being normalization of oxygenation, shunt, and alveolar-arterial oxygen gradient. Their study demonstrated worse survival in the ventilated group owing to the complications of mechanical ventilation. Shackford et al. concluded that mechanical ventilation should be used to correct abnormalities of gas exchange rather than to overcome instability of the chest wall. In a prospective study several years later, Shackford et al. divided patients with FC (n = 36) by severity of injury and provided ventilatory support only when a clinical indication developed.[19] Outcomes were compared with historical controls. Overall ventilatory rates decreased from 74% to 38% (p < 0.01) from the previous study and mortality from 14% to 8%, (p < 0.01). Other recent studies have supported the selective use of ventilatory support for defects in gas exchange and clinical indications only rather than for correction of mechanical abnormalities of the chest wall.[5][20][21] Studies addressing decision for ventilatory support are reviewed and graded in the evidentiary tables.
Modes of Ventilatory Support
As early as 1972, McGee and Trinkle[73] clearly demonstrated that the size of experimental PC s in dogs was significantly decreased by the applications of PEEP. The initial prospective human study by Sladen et al.[22] involved varying levels of PEEP in a small group of patients (n = 9) who served as their own controls. Despite the small study size, PO2 improved to a significant degree in all patients with PEEP of 10 cm or 15 cm of water. There was no change in physiologic dead space, and therefore the improvements were attributed to alveolar “recruitment” or increased FRC. Rib fracture alignment was anecdotally noted to be improved on fluoroscopy, but the significance of this was not addressed. Survival benefit could not be assessed because this was a single-arm study.
Only occasional work has addressed the actual choice of ventilatory modes for PC-FC injuries. In the salient work on this issue, Pinella[23] studied the use of intermittent mandatory ventilation (IMV) in 144 patients with varying severity of FC against historical controls on continuous mandatory ventilation. Groups were well matched in terms of severity of flail and associated injuries. No difference could be identified in terms of duration of ventilatory support, level of PEEP or FIO2, or outcome between the continuous mandatory ventilation and IMV group.
Recent attention has focused on the use of CPAP modes both noninvasively and by endotracheal intubation.[24][25] The critical animal study by Schweiger et al.[25]compared IMV to CPAP in three groups of pigs: a control group, FC group, and PC-FC group. Ten centimeters to fifteen centimeters of CPAP was beneficial over IMV alone for correcting alveolar closure, thereby minimizing shunt fraction (p < 0.001) and improving compliance significantly (p < 0.006). The need for IMV was significantly reduced after the application of CPAP in all animals (p < 0.01). This effect was more pronounced in PC-FC than in isolated FC (p < 0.01). Similarly, in humans, Tanaka et al.[24] prospectively studied the use of noninvasive CPAP in 59 patients with FC injury (Class II). Study patients were compared with historical controls treated for respiratory failure primarily with mechanical ventilation. Groups were well matched in terms of extent of chest wall injury and overall injury severity. The CPAP group had a lower rate of pulmonary complications (atelectasis, 47% vs. 95%; pneumonia, 27% vs. 70%; p < 0.01) and a significantly lower rate of mechanical ventilation. More recently, similar results were obtained by Gunduz et al., who conducted a prospective randomized study (n = 25 in each limb) of CPAP with patient-controlled analgesia versus endotracheal intubation for patients with FC injury (Level I). Results showed that survival and nosocomial infection rates were significantly lower in the CPAP group (p < 0.001)
For completeness, mention of HFOV should be made. While the literature is replete with studies suggesting benefit in established adult and pediatric adult respiratory distress syndrome (ARDS),[74–85] the use of this modality for primary PC has only been minimally studied. Since patients with PC are thought to be more likely to develop ARDS and since HFOV theoretically achieves all the goals of the lung protective strategy of ARDSNet,[74][86–88] it has been hypothesized that HFOV might be useful in severe PC. Funk et al.[89] retrospectively studied 17 patients for whom the use of HFOV for PC was “activated” by protocol when peak airway pressure was greater than 30 cm and FIO2 was greater than 60%. This review showed a statistically significant improvement in PaO2/FIO2 ratio. Mortality was less than predicted by Acute Physiology and Chronic Health Evaluation (APACHE) score and Trauma and Injury Severity Score (TRISS). However, no comparison was made with the aggressive use of conventional ventilation.
Finally, independent lung ventilation (ILV) has been used sporadically during the last 20 years. This modality has been applied to patients with severe unilateral chest trauma, predominantly PC in whom major ventilation-perfusion mismatch has been unresponsive to conventional support. Most of the work on this modality has consisted of case reports[90–96] or small, uncontrolled, single-limb observational studies,[97–99] which report improved oxygenation and survival for patients who were failing conventional ventilation. The rationale for ILV rests with the supposition that the severe ventilation-perfusion mismatch of extensive PC is worsened by the asymmetrical compliance of the injured lung.[90] This occurs through diversion of ventilation to more compliant areas causing overdistention of normal alveoli. Hurst et al.[99] initiated ILV for eight patients with unilateral PC with and without FC who were failing conventional support. Significant improvements were obtained in PaO2(72 + 8.7 to 153 + 37; p < 0.005) and shunt fraction (28 + 3.5 to 12.6 + 2.5; p < 0.005). No significant changes occurred in cardiac output, peripheral resistance, or oxygen extraction index. Seven of the eight patients survived. Although this study was prospective, selection was nonrandom, and no control group was studied (Class II). Studies addressing modes of ventilatory support are reviewed and graded in the evidentiary tables.
Surgical Repair of FC/Rib Fractures
Surgical stabilization of FC injury has been used with some frequency in Europe and Asia from the 1950s until present day.[47][100–108].Numerous European studies reported “good” results with surgical fixation of FC, citing decreased pain, improved mechanics compared with preoperative performance, “rapid” separation from mechanical ventilation, and excellent return-to-work outcomes. Yet, these studies are mostly small, single-limb, observational studies of personal experience lacking nonsurgical controls (Classes II and III).[47][100–102][109–113] In some, patient selection is nonrandom.[103][104][111–115]
Tanaka et al.[116] performed the salient randomized, controlled study (Class I) of operative fixation versus obligatory internal pneumatic stabilization. Groups (n = 37) were well matched in terms of injury severity, criteria for ventilatory support, and ventilator management. The incidence of pneumonia was less in the surgical group (22% vs. 90%) as was the length of ventilation and length of intensive care unit (ICU) stay. The investigators reported improved lung volumes, decreased pain and dyspnea, and higher return-to-work at 1 year with surgical fixation. All findings were significant at p < 0.05. Tanaka et al. concluded that surgical stabilization may be preferable for patients with severe FC when prolonged ventilatory support would otherwise be expected. In a similar, but retrospective review of 64 patients, Balci et al.[115] also compared operative fixation with ventilator support. The surgical group had a lower mortality (11% vs. 21%), less ventilator days (3 vs. 6.6), and less narcotic use. However, patient allocation was not randomized in this study. Finally, Voggenreiter et al.[117] compared the outcome of operative fixation for FC alone and flail with PC to a nonoperative control group. Groups were well matched. “Pure” FC patients benefited from surgical fixation in terms of separation from mechanical ventilation (6.5 vs. 30 days; p < 0.02), while those with PC-FC did not (27 vs. 30 days). These authors concluded that FC and respiratory insufficiency without underlying PC is an indication for surgical fixation. They thought that the presence of PC-FC precludes benefit from primary fixation, but that secondary stabilization may be indicated in the weaning period. This study was uncontrolled and retrospective and involved a small sample size.
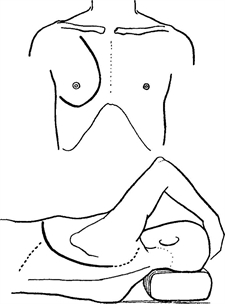
Figure 2.
Recently more experience has been accrued in the United States.[118–123] However, most studies continue to be single-limb retrospective series[122][123] (Grade III evidence) or prospective studies against historical controls[119][121] (Grade II evidence). In one of the few well-designed Level II studies, Nirula et al.[119] prospectively studied 30 consecutive patients with repair of FC using strut fixation versus a matched set of historical controls. LOS and ICU LOS were the same. There was a trend toward fewer ventilator days for the surgical group and total ventilator days after fixation was shorter than total ventilator days for the conservative group (2.9 [0.6] vs. 9.4 [2.7]). Patients were not randomly selected, and total ventilator days were not significantly different, but the rapid wean after fixation does suggest a benefit. Similarly, Marasco et al.[121] showed decreased mortality in patient with fixated FC (0 of 13) versus controls (22 of 106). However, sample size was small, and controls were historical.
No prospective, randomized controlled studies were identified comparing surgical fixation to modern conservative treatment with epidural analgesia and chest physiotherapy. Consequently, although surgical fixation clearly corrects the anatomic chest deformity, comparison of its efficacy with that of conservative treatment remains problematic.[103] In a recent survey of trauma surgeons, 90% indicated that they would not adopt the practice of surgical rib fixation until adequate data from randomized controlled trials were available.[122]
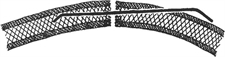
Figure 3.
Surgical stabilization of multiple rib fractures historically involves a significant operative procedure with mobilization of large chest wall flaps or open thoracotomy[47](Fig. 2). More recently, less invasive procedures have been used using several smaller incisions with computed tomography–guided reconstructions or real-time thoracoscopic monitoring serving as a road map.[120][121]
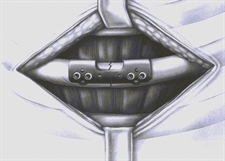
Figure 4.
A variety of generic orthopedic devices have been used to stabilize the fracture fragments including medullary wires or nails, Judet struts, or compression plates[47][101][102][104][110][111] (Fig. 3). More recently a number “rib-specific” proprietary devices have been developed including rib “splints” (MatrixRIB, Synthes CMF, West Chester, PA)[124], a mesh wrap (Inion Orthopedic Trauma Plating System, Inion Oy, Tampere, Finland)[120] and a U-plate fixation system (“Ribloc,” Acute Innovations, Hillsboro, OR) (Fig. 4).[125] Each device is promoted by its manufacturer as providing greater ease of placement and more durable fixation and have been subjected to third-party in-vitro biomechanical testing against traditional plates and intramedullary devices, but not against each other.[124–127]Consequently, the ideal rib fracture fixation device remains to be identified. Biomechanical testing by several groups does seen to indicate that some type of external plate or splint is most likely more durable than intramedullary wires with greater load tolerance and less tendency to fail catastrophically with loss of fixation.[124–127]
Specifics of the operative technique are beyond the scope of this review and the reader is referred to specific reports on the subject.[62][105][106][108][114][115] Available literature addressing surgical fixation of FC is reviewed and graded in the evidentiary table.
Other Miscellaneous Therapies
Steroids
The use of steroids for the treatment of PC has rarely been addressed in the literature. Franz et al.[26] administered methylprednisolone 30 minutes after creation of experimental PC in dogs. The weight ratio of contused to normal lung was significantly decreased in treated animals, and the volume of injury was less on post mortem (p < 0.05). Since the animals were killed, the effect of steroids on recovery and survival could not be assessed. In a small retrospective human study, Svennevig et al.[128] concluded that the mortality in severe chest injury was reduced through the use of steroids. This study, however, involved neither randomization nor constant criteria for administration of steroids. Since the cause of deaths were not specified, it was difficult to assess the complications and risk versus benefit of steroid use.
Multidisciplinary Clinical Pathways
Clinical pathways have been shown to improve LOS and have been developed for many diagnoses in numerous specialties. Recently, Todd et al.[129] tested a “self-activating” multidisciplinary clinical pathway for rib fracture management. Patients of age greater than 45 years with four-rib or more rib fractures were identified and monitored early on. Analog pain scale greater than 6, incentive spirometry less than 15 mL/kg, and subjective assessment of weak cough triggered an aggressive multidisciplinary treatment by nursing, pain service, and respiratory therapy. This prospective Level II study used historical controls (n = 150 in each limb) but showed a very significant decrease in ICU and hospital LOS (p < 0.02) and in incidence of pneumonia (p < 0.001) There was a trend toward decreased mortality. This study was well powered and gave Level II validity to what is empirically known, that early use of aggressive, attentive care for select patients will improve outcomes.
Newer Modalities of Pain Control
The options and controversies regarding pain control in chest wall injury are beyond the scope of this study, and the reader is referred to the EAST guideline on Analgesia in Blunt Thoracic Trauma.[130] However, mention should be made of the increased use of local anesthetic administered by transcutaneous patch (lidocaine 5%, Lidoderm, Endo Pharmaceuticals) since that guideline was published. At this writing, the patch is Food and Drug Administration approved for postherpetic neuralgia, but it has been used “off-label” for other analgesic indications including chronic lower back pain,[131] postlaparoscopic surgical pain,[132] and headache. Ingalls et al.[133] performed an eloquent prospective, randomized controlled trial of lidocaine 5% patch versus placebo for patients with rib fractures. The study was well powered, and the lidocaine patch failed to show sparing of narcotics versus the placebo group. Yet, other work in progress may indicate a narcotic-sparing effect of the patch (unpublished data, work in progress).
Vasopressin for PC
Arginine vasopressin versus placebo was assessed in the resuscitation of pulmonary contused pigs that were subjected to controlled and uncontrolled hemorrhage and then crystalloid resuscitation.[134] In controlled hemorrhage, there was higher mortality without arginine vasopressin, more fluid requirement, and increased peak airway pressures and p/f ratios. In uncontrolled hemorrhage model, there was a trend toward increased survival and reduced blood loss. Although this was an animal study and not definitive, further work may be warranted on this treatment.
“Other miscellaneous therapies” are tabulated in the evidentiary tables.
Conclusion
PC-FC is a common injury constellation in blunt trauma. While injuries to the chest wall itself may rarely be the primary cause of death in patients with multiple injuries, they greatly impact management, survival, and long-term disability. When occurring in sufficient volume of the lung, PC may have adverse global pulmonary and systemic effects.
Most of the current practice in treatment of PC-FC derives from a modest quantity of Class II and III work, extrapolation of animal research, and “local custom.” There is currently no credible human evidence that “fluid restriction” improves outcome, although it has been shown to improve oxygenation in animal models. Respiratory dysfunction after contusion may ultimately be shown to relate more to direct traumatic and indirect biochemical effects of the injury rather than amounts of fluid administered. In terms of ventilatory management, the bulk of current evidence favors selective use of mechanical ventilation with analgesia and chest physiotherapy being the preferred initial strategy. When support is required, no specific mode has been shown to be superior to others, although there is reasonable evidence that addition of PEEP or CPAP is helpful in improving oxygenation. While the literature supporting the use of independent lung ventilation in severe unilateral PC is largely observational, most of the work supports the opinion that it may be beneficial in select patients. Finally, surgical fixation of FC has not been credibly compared with modern selective management but may also be a valuable addition to the armamentarium in appropriate circumstances.
Areas for Further Investigation
Significant quantitative and qualitative gaps exist in the body of knowledge regarding PC-FC. Areas in need of further investigation include the following:
- Effect of hypertonic saline resuscitation on PC
- Newer modes of regional analgesia
- Anti-inflammatory “anti-cytokine” Rx
- Modes of ventilatory support
- Noninvasive ventilatory support
- Indications and techniques of surgical fixation
- Long-term outcomes.
Disclosure
The authors declare no conflicts of interest.
References
- Allen GS, Coates NE. Pulmonary contusion: a collective review. Am Surg. 1996; 62: 895–900.
- Taylor GA, et al.. Controversies in the management of pulmonary contusion. Can J Surg. 1982; 25: 167–170.
- Clark GC, Schecter WP, Trunkey DD. Variables affecting outcome in blunt chest trauma: flail chest vs. pulmonary contusion. J Trauma. 1988; 28: 298–304.
- Glinz W. Problems caused by the unstable thoracic wall and by cardiac injury due to blunt injury. Injury. 1986; 17: 322–326.
- Miller HA, et al.. Management of flail chest. Can Med Assoc J. 1983; 129: 1104–1107.
- Richardson JD, Adams L, Flint LM. Selective management of flail chest and pulmonary contusion. Ann Surg. 1982; 196: 481–487.
- Trinkle JK, et al.. Management of flail chest without mechanical ventilation. Ann Thorac Surg. 1975; 19: 355–363.
- Albaugh G, et al.. Age-adjusted outcomes in traumatic flail chest injuries in the elderly. Am Surg. 2000; 66: 978–981.
- Adegboye VO, et al.. Blunt chest trauma. Afr J Med Med Sci. 2002; 31: 315–320.
- Kishikawa M, et al.. Pulmonary contusion causes long-term respiratory dysfunction with decreased functional residual capacity. J Trauma. 1991; 31: 1203–1208; discussion 1208–1210.
- Beal SL, Oreskovich MR. Long-term disability associated with flail chest injury. Am J Surg. 1985; 150: 324–326.
- Christensson P, et al.. Early and late results of controlled ventilation in flail chest. Chest. 1979; 75: 456–460.
- Shackford SR, et al.. The management of flail chest. A comparison of ventilatory and nonventilatory treatment. Am J Surg. 1976; 132: 759–762.
- Fulton RL, Peter ET. Physiologic effects of fluid therapy after pulmonary contusion. Am J Surg. 1973; 126: 773–777.
- Trinkle JK, et al.. Pulmonary contusion. Pathogenesis and effect of various resuscitative measures. Ann Thorac Surg. 1973; 16: 568–573.
- Bongard FS, Lewis FR. Crystalloid resuscitation of patients with pulmonary contusion. Am J Surg. 1984; 148: 145–151.
- Johnson JA, Cogbill TH, Winga ER. Determinants of outcome after pulmonary contusion. J Trauma. 1986; 26: 695–697.
- Cohn SM, et al.. Resuscitation of pulmonary contusion: effects of a red cell substitute. Crit Care Med. 1997; 25: 484–491.
- Shackford SR, Virgilio RW, Peters RM. Selective use of ventilator therapy in flail chest injury. J Thorac Cardiovasc Surg. 1981; 81: 194–201.
- Carpintero JL, et al.. Methods of management of flail chest. Intensive Care Med. 1980; 6: 217–221.
- Dittmann M, et al.. Epidural analgesia or mechanical ventilation for multiple rib fractures? Intensive Care Med. 1982; 8: 89–92.
- Sladen A, Aldredge CF, Albarran R. PEEP vs. ZEEP in the treatment of flail chest injuries. Crit Care Med. 1973; 1: 187–191.
- Pinella J. Acute respiratory failure in severe blunt chest trauma. J Trauma. 1982; 22: 221–225.
- Tanaka H, et al.. Pneumatic stabilization for flail chest injury: an 11-year study. Surg Today. 2001; 31: 12–17.
- Schweiger JW, Downs JB, Smith RA. Chest wall disruption with and without acute lung injury: effects of continuous positive airway pressure therapy on ventilation and perfusion relationships. Crit Care Med. 2003; 31: 2364–2370.
- Franz JL, et al.. Effect of methylprednisolone sodium succinate on experimental pulmonary contusion. J Thorac Cardiovasc Surg. 1974; 68: 842–844.
- Odelowo EO. Successful management of flail chest without the use of volume ventilator. East Afr Med J. 1987; 64: 836–844.
- Rouby JJ, et al.. Continuous positive airway pressure (CPAP) vs. intermittent mandatory pressure release ventilation (IMPRV) in patients with acute respiratory failure. Intensive Care Med. 1992; 18: 69–75.
- Velmahos GC, et al.. Influence of flail chest on outcome among patients with severe thoracic cage trauma. Int Surg. 2002; 87: 240–244.
- Jadad AR, McQuay HJ. Searching the literature. Be systematic in your searching. BMJ. 1993; 307: 66.
- Lockwood AL. Some experiences in the last war. BMJ. 1940; 1: 356–358.
- Thomson FG. Penetrating chest wounds. BMJ. 1940; 1: 44–46.
- Kretzschmar CH. Wounds of the chest treated by artificial pneumothorax. Lancet. 1940; 1: 832.
- Osborn H, et al.. Biological abstracts. Science. 1924; 60: 485–489.
- Zuckerman S. Experimental study of blast injury to the lungs. Lancet. 1940; 2: 219.
- Desaga H. Blast Injuries. German Aviation Med WWII. Washington, DC: U.S. Government Printing Office; 1950.
- Cameron AE. War and health of animals division. Can J Comp Med Vet Sci. 1942; 6: 46–49.
- Clark CW. Traumatic arterial spasm. BMJ. 1943; 2: 167.
- Burford TH, Burbank B. Traumatic wet lung; observations on certain physiologic fundamentals of thoracic trauma. J Thorac Surg. 1945; 14: 415–424.
- Brewer LA, Burbank B, et al.. The wet lung in war casualties. Ann Surg. 1946; 123: 343–362.
- Moseley RV, Doty DB, Pruitt BA Jr. Physiologic changes following chest injury in combat casualties. Surg Gynecol Obstet. 1969; 129: 233–242.
- Lichtmann MW. The problem of contused lungs. J Trauma. 1970; 10: 731–739.
- Ratliff JL, et al.. Pulmonary contusion. A continuing management problem. J Thorac Cardiovasc Surg. 1971; 62: 638–644.
- Reid JM, Baird WL. Crushed chest injury: some physiological disturbances and their correction. BMJ. 1965; 1: 1105–1109.
- Wilkinson A. The management of 220 cases of flail chest injuries. S Afr J Surg. 1977; 15: 21–30.
- Avery EE, Benson DW, Morch ET. Critically crushed chests; a new method of treatment with continuous mechanical hyperventilation to produce alkalotic apnea and internal pneumatic stabilization. J Thorac Surg. 1956; 32: 291–311.
- Moore BP. Operative stabilization of nonpenetrating chest injuries. J Thorac Cardiovasc Surg. 1975; 70: 619–630.
- Diethelm AG, Battle W. Management of flail chest injury: a review of 75 cases. Am Surg. 1971; 37: 667–670.
- Garzon AA, Seltzer B, Karlson KE. Physiopathology of crushed chest injuries. Ann Surg. 1968; 168: 128–136.
- Oppenheimer L, et al.. Pathophysiology of pulmonary contusion in dogs. J Appl Physiol. 1979; 47: 718–728.
- Hellinger A, et al.. Does lung contusion affect both the traumatized and the noninjured lung parenchyma? A morphological and morphometric study in the pig. J Trauma. 1995; 39: 712–719.
- Fulton RL, Peter ET. The progressive nature of pulmonary contusion. Surgery. 1970; 67: 499–506.
- Craven KD, Oppenheimer L, Wood LD. Effects of contusion and flail chest on pulmonary perfusion and oxygen exchange. J Appl Physiol. 1979; 47: 729–737.
- Wagner RB, et al.. Effect of lung contusion on pulmonary hemodynamics. Ann Thorac Surg. 1991; 52: 51–57; discussion 57–58.
- Davis KA, et al.. Prostanoids: early mediators in the secondary injury that develops after unilateral pulmonary contusion. J Trauma. 1999; 46: 824–831; discussion 831–832.
- Perl M, et al.. Pulmonary contusion causes impairment of macrophage and lymphocyte immune functions and increases mortality associated with a subsequent septic challenge. Crit Care Med. 2005; 33: 1351–1358.
- Hoth JJ, et al.. Toll-like receptor 2 participates in the response to lung injury in a murine model of pulmonary contusion. Shock. 2007; 28: 447–452.
- Hoth JJ, et al.. Pulmonary contusion primes systemic innate immunity responses. J Trauma. 2009; 67: 14–21; discussion 21–22.
- Hoth JJ, et al.. Toll-like receptor 4-dependent responses to lung injury in a murine model of pulmonary contusion. Shock. 2009; 31: 376–381.
- Balci AE, et al.. Unilateral post-traumatic pulmonary contusion: findings of a review. Surg Today. 2005; 35: 205–210.
- Amital A, et al.. Long-term pulmonary function after recovery from pulmonary contusion due to blunt chest trauma. Isr Med Assoc J. 2009; 11: 673–676.
- Hamrick MC, Duhn RD, Ochsner MG. Critical evaluation of pulmonary contusion in the early post-traumatic period: risk of assisted ventilation. Am Surg. 2009; 75: 1054–1058.
- Deunk J, et al.. The clinical outcome of occult pulmonary contusion on multidetector-row computed tomography in blunt trauma patients. J Trauma. 2010; 68: 387–394.
- Kollmorgen DR, et al.. Predictors of mortality in pulmonary contusion. Am J Surg. 1994; 168: 659–663; discussion 663–664.
- Stellin G. Survival in trauma victims with pulmonary contusion. Am Surg. 1991; 57: 780–784.
- Relihan M, Litwin MS. Morbidity and mortality associated with flail chest injury: a review of 85 cases. J Trauma. 1973; 13: 663–671.
- Flagel BT, et al.. Half-a-dozen ribs: the breakpoint for mortality. Surgery. 2005; 138: 717–723; discussion 723–725.
- Borman JB, et al.. Unilateral flail chest is seldom a lethal injury. Emerg Med J. 2006; 23: 903–905.
- Bakhos C, et al.. Vital capacity as a predictor of outcome in elderly patients with rib fractures. J Trauma. 2006; 61: 131–134.
- Landercasper J, Cogbill TH, Lindesmith LA. Long-term disability after flail chest injury. J Trauma. 1984; 24: 410–414.
- Kishikawa M, et al.. Laterality of air volume in the lungs long after blunt chest trauma. J Trauma. 1993; 34: 908–912; discussion 912–913.
- Richardson JD, et al.. Pulmonary contusion and hemorrhage—crystalloid versus colloid replacement. J Surg Res. 1974; 16: 330–336.
- McGee EM, Trinkle JK. Pulmonary contusion—pathogenesis and current management. Rev Surg. 1972; 29: 224.
- Treggiari MM, et al.. Effect of acute lung injury and acute respiratory distress syndrome on outcome in critically ill trauma patients. Crit Care Med. 2004; 32: 327–331.
- Gerstmann DR, et al.. The Provo multicenter early high-frequency oscillatory ventilation trial: improved pulmonary and clinical outcome in respiratory distress syndrome. Pediatrics. 1996; 98 (6 pt 1): 1044–1057.
- Arnold JH, et al.. Prospective, randomized comparison of high-frequency oscillatory ventilation and conventional mechanical ventilation in pediatric respiratory failure. Crit Care Med. 1994; 22: 1530–1539.
- Chan KP, Stewart TE. Clinical use of high-frequency oscillatory ventilation in adult patients with acute respiratory distress syndrome. Crit Care Med. 2005; 33 (3 suppl): S170–S174.
- Derdak S, et al.. High-frequency oscillatory ventilation for acute respiratory distress syndrome in adults: a randomized, controlled trial. Am J Respir Crit Care Med. 2002; 166: 801–808.
- Bernard GR, et al.. Report of the American-European Consensus conference on acute respiratory distress syndrome: definitions, mechanisms, relevant outcomes, and clinical trial coordination. Consensus Committee. J Crit Care. 1994; 9: 72–81.
- Fort P, et al.. High-frequency oscillatory ventilation for adult respiratory distress syndrome—a pilot study. Crit Care Med. 1997; 25: 937–947.
- Mehta S, et al.. Prospective trial of high-frequency oscillation in adults with acute respiratory distress syndrome. Crit Care Med. 2001; 29: 1360–1369.
- Cartotto R, et al.. Early clinical experience with high-frequency oscillatory ventilation for ARDS in adult burn patients. J Burn Care Rehabil. 2001; 22: 325–333.
- Claridge JA, et al.. High-frequency oscillatory ventilation can be effective as rescue therapy for refractory acute lung dysfunction. Am Surg. 1999; 65: 1092–1096.
- Andersen FA, Guttormsen AB, Flaatten HK. High frequency oscillatory ventilation in adult patients with acute respiratory distress syndrome—a retrospective study. Acta Anaesthesiol Scand. 2002; 46: 1082–1088.
- Kao KC, et al.. High frequency oscillatory ventilation for surgical patients with acute respiratory distress syndrome. J Trauma. 2006; 61: 837–843.
- Pepe PE, et al.. Clinical predictors of the adult respiratory distress syndrome. Am J Surg. 1982; 144: 124–130.
- Croce MA, et al.. Early and late acute respiratory distress syndrome: two distinct clinical entities. J Trauma. 1999; 46: 361–366; discussion 366–368.
- Freedland M, et al.. The management of flail chest injury: factors affecting outcome. J Trauma. 1990; 30: 1460–1468.
- Funk DJ, et al.. A brief report: the use of high-frequency oscillatory ventilation for severe pulmonary contusion. J Trauma. 2008; 65: 390–395.
- Katsaragakis S, Stamou KM, Androulakis G. Independent lung ventilation for asymmetrical chest trauma: effect on ventilatory and haemodynamic parameters.Injury. 2005; 36: 501–504.
- Ost D, Corbridge T. Independent lung ventilation. Clin Chest Med. 1996; 17: 591–601.
- Miller RS, et al.. Synchronized independent lung ventilation in the management of a unilateral pulmonary contusion with massive hemoptysis. J Tenn Med Assoc. 1992; 85: 374–375.
- Watts DC, et al.. Pressure-controlled inverse-ratio synchronised independent lung ventilation for a blast wound to the chest. Clin Intensive Care. 1991; 2: 356–358.
- Wendt M, et al.. Differential ventilation with low-flow CPAP and CPPV in the treatment of unilateral chest trauma. Intensive Care Med. 1989; 15: 209–211.
- Frame SB, Marshall WJ, Clifford TG. Synchronized independent lung ventilation in the management of pediatric unilateral pulmonary contusion: case report. J Trauma. 1989; 29: 395–397.
- Albert J, Varraux AR. Independent lung ventilation. Crit Care Med. 1981; 9: 131–132.
- Cinnella G, et al.. Independent lung ventilation patients with unilateral pulmonary contusion. Monitoring with compliance and EtCO. Intensive Care Med. 2001; 27: 1860–1867.
- Crimi G, et al.. Clinical applications of independent lung ventilation with unilateral high-frequency jet ventilation (ILV-UHFJV). Intensive Care Med. 1986; 12: 90–94.
- Hurst JM, DeHaven CB Jr, Branson RD. Comparison of conventional mechanical ventilation and synchronous independent lung ventilation (SILV) in the treatment of unilateral lung injury. J Trauma. 1985; 25: 766–770.
- Hellberg K, et al.. Stabilization of flail chest by compression osteosynthesis—experimental and clinical results. Thorac Cardiovasc Surg. 1981; 29: 275–281.
- Sanchez-Lloret J, et al.. Indications and surgical treatment of the traumatic flail chest syndrome. An original technique. Thorac Cardiovasc Surg. 1982; 30: 294–297.
- Reber P, et al.. Osteosynthesis of the injured chest wall. Use of the AO (Arbeitsgemeinschaft fur Osteosynthese) technique. Scand J Thorac Cardiovasc Surg. 1993; 27: 137–142.
- Ahmed Z, Mohyuddin Z. Management of flail chest injury: internal fixation versus endotracheal intubation and ventilation. J Thorac Cardiovasc Surg. 1995; 110: 1676–1680.
- Lardinois D, et al.. Pulmonary function testing after operative stabilisation of the chest wall for flail chest. Eur J Cardiothorac Surg. 2001; 20: 496–501.
- Iwasaki Y., et al.. Surgical stabilization of multiple rib fracture and flail chest [in Japanese]. Kyobu Geka. 2006; 59: 980–984.
- Kakegawa S, et al.. Surgical fixation of the ribs for flail chest injuries [in Japanese]. Kyobu Geka. 2006; 59: 974–979.
- Vodicka J, et al.. Reconstruction of a crushed chest with HI-TEX PARP NT implant [in German]. Unfallchirurg. 2007; 110: 255–258.
- Zhu HH, et al.. Treatment of fracture of multiple ribs with absorbable rib fixed nail and dacron flap in 12 patients [in Chinese]. Zhongguo Gu Shang. 2009; 22: 787–789.
- Paris F, et al.. Surgical stabilization of traumatic flail chest. Thorax. 1975; 30: 521–527.
- Thomas AN, et al.. Operative stabilization for flail chest after blunt trauma. J Thorac Cardiovasc Surg. 1978; 75: 793–801.
- Menard A, et al.. Treatment of flail chest with Judet’s struts. J Thorac Cardiovasc Surg. 1983; 86: 300–305.
- Mouton W, et al.. Long-term follow-up of patients with operative stabilisation of a flail chest. J Thorac Cardiovasc Surg. 1997; 45: 242–244.
- Mayberry JC, et al.. Absorbable plates for rib fracture repair: preliminary experience. J Trauma. 2003; 55: 835–839.
- Galan G, et al.. Blunt chest injuries in 1696 patients. Eur J Cardiothorac Surg. 1992; 6: 284–287.
- Balci AE, et al.. Open fixation in flail chest: review of 64 patients. Asian Cardiovasc Thorac Ann. 2004; 12: 11–15.
- Tanaka H, et al.. Surgical stabilization of internal pneumatic stabilization? A prospective randomized study of management of severe flail chest patients. J Trauma. 2002; 52: 727–732; discussion 732.
- Voggenreiter G, et al.. Operative chest wall stabilization in flail chest–outcomes of patients with or without pulmonary contusion. J Am Coll Surg. 1998; 187: 130–138.
- Engel C. Operative fixation with osteosynthesis plates. J Trauma. 2006; 58: 181–186.
- Nirula R, et al.. Rib fracture stabilization in patients sustaining blunt chest injury. Am Surg. 2006; 72: 307–309.
- Campbell N, et al.. Surgical stabilization of rib fractures using Inion OTPS wraps—techniques and quality of life follow-up. J Trauma. 2009; 67: 596–601.
- Marasco S, et al.. Pilot study of operative fixation of fractured ribs in patients with flail chest. ANZ J Surg. 2009; 79: 804–808.
- Mayberry JC, et al.. Surveyed opinion of American trauma, orthopedic, and thoracic surgeons on rib and sternal fracture repair. J Trauma. 2009; 66: 875–879.
- Solberg BD, et al.. Treatment of chest wall implosion injuries without thoracotomy: technique and clinical outcomes. J Trauma. 2009; 67: 8–13; discussion 13.
- Helzel I. Evaluation of intramedullary rib splints for less-invasive stabilization of rib fractures. Injury. 2009; 40: 1104–1110.
- Sales JR, et al.. Biomechanical testing of a novel, minimally invasive rib fracture plating system. J Trauma. 2008; 64: 1270–1274.
- Bottlang M, et al.. Less-invasive stabilization of rib fractures by intramedullary fixation: a biomechanical evaluation. J Trauma. 2010; 68: 1218–1224.
- Campbell N, Richardson M, Antippa P. Biomechanical testing of two devices for internal fixation of fractured ribs. J Trauma. 2010; 68: 1234–1238.
- Svennevig JL, et al.. Early use of corticosteroids in severe closed chest injuries: a 10-year experience. Injury. 1987; 18: 309–312.
- Todd SR, et al.. A multidisciplinary clinical pathway decreases rib fracture-associated infectious morbidity and mortality in high-risk trauma patients. Am J Surg. 2006; 192: 806–811.
- Simon BJ, et al.. Pain management guidelines for blunt thoracic trauma. J Trauma. 2005; 59: 1256–1267.
- Gimbel J, et al.. Lidocaine patch treatment in patients with low back pain: results of an open-label, nonrandomized pilot study. Am J Ther. 2005; 12: 311–319.
- Saber AA, et al.. Early experience with lidocaine patch for postoperative pain control after laparoscopic ventral hernia repair. Int J Surg. 2009; 7: 36–38.
- Ingalls NK, et al.. Randomized, double-blind, placebo-controlled trial using lidocaine patch 5% in traumatic rib fractures. J Am Coll Surg. 2010; 210: 205–209.
- Feinstein AJ, et al.. Early vasopressin improves short-term survival after pulmonary contusion. J Trauma. 2005; 59: 876–882; discussion 882–883.
Table
Table 1. Reported Physiologic Effects of Lung Contusion
Local Effects |
Laceration to lung tissue Hemorrhage-filled alveoli Reduced compliance yielding reduced ventilation Increased shunt fraction with decrease in PO2, increase in AaDO2 Increased pulmonary vascular resistance Decreased pulmonary blood flow |
Injured and uninjured lung (ipsilateral and contralateral) Thickened alveolar septa with impaired diffusion Decreased alveolar diameter Vacuolation of pulmonary tissue Delayed capillary leak with increased BAL protein Increased neutrophils in lung tissue |
Systemic Increased TCC Decreased complement Increased cytokines tumor necrosis factor > and interleukin 6 Decreased peritoneal macrophase, splenic macrophage, and splenocytes Increased mortality for a septic challenge |
AaDO2, alveolar-arterial oxygen difference; TCC, terminal complement component. |